The Industry of Plant Breeding
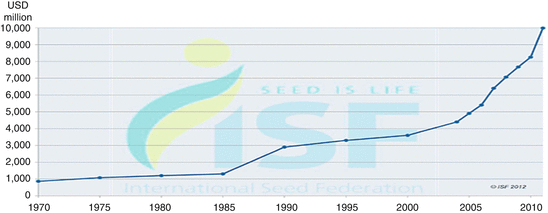
(1)
Max Planck Institute for Innovation and Competition, Munich, Germany
3.1 The Significance of Plant Breeding in Society
Plant breeding aims at creating useful plants for the benefit of humankind. At the heart of these activities, stands the knowledge of the art and science of ameliorating plant genetics.1 The amelioration of the genetics of plants dates back to the Neolithic Age, when most of the major cereals, legumes and root crops where successfully domesticated.2 These crops continue to be man’s predominant source of food, although their production has undergone through continuous changes to enhance the quantity and quality of their yields. Initially, the improvement of plants was a communal activity. It relied on the knowledge that individuals and indigenous communities had acquired over time from one generation to another. Unaware of the scientific aspects inherent in plant amelioration, they based the selection and the creation of plants with desired properties on the characteristics of the phenotypes that could be observed with the naked eye.
While the principals of these ancient practices remain to this day an important foundation for plant breeding, breeding methods have substantially changed through time.3 This is certainly due to the progress of science. Nowadays, plant breeding relies on the methods and techniques of applied genetics, usually combined with molecular biology, cytology, systematics, physiology, pathology, entomology, chemistry, and statistics, as well as breeding techniques recently developed in plant biotechnology.4
The application of these scientific methods in plant breeding brings about considerable benefits for humankind. For instance, the possibility to obtain food with high nutritional qualities from modified plants is one of the greatest achievements of plant breeding in improving life quality and combating hunger. Besides food production, improved plants can be further employed in agriculture, industry and forestry to provide feed for livestock, fiber for clothing and furnishing, and fuel for cooking and heating. Employing modern plants in various industrial sectors does not only increase production and economic profits, but it supports sustainable development as well. This role of plant breeding will be further illustrated in the following paragraphs. Afterward, the evolution of the plant breeding industry will be briefly explained. This will provide the reader with a first understanding of plant breeding.
3.1.1 Plant Breeding and Food Production
Without exception, the main importance of plant breeding is directly perceived in food production. The urge for food supply arises from the constant growth of the world population which is expected to exceed nine billion by 2050. Providing food for the world population was also part of the objectives of the so-called ‘green revolution’5 Between the 1960s and the 1990s, the ‘green revolution’, supported by various agricultural centers operating under the auspices of the Consultative Group for International Agricultural Research (CGIAR), promoted the diffusion of modern varieties both in the developed and developing countries.6 The results were remarkable. The major plant breeding breakthroughs were for maize and wheat. For example, wheat production more than tripled between the late 1960s and the mid-1980s in India. This represented sufficient grain to satisfy the dietary needs of 186 million people. In addition, new crop species with enhanced qualities were developed. This is the case of triticale—a crossing of wheat and rye—which has higher yield advantages over wheat.7
This enormous progress has lately been furthered by employing genetic modification (GM) techniques in plant breeding. These techniques propone to alleviate the problem of food insecurity and environmental degradation often caused by the intensive use of pesticides and fertilizers during the ‘green revolution’ by enhancing the nutritional content of food, reducing the use of pesticides, and improving crops resistance to draught, heat, frost and soil salinity.8 The potential of this new technology in reducing poverty and hunger in the world is, however, controversial. Some studies have found a statistically significant relationship between increased crop yields and increased adoption of herbicide and pesticide tolerant crop seeds,9 whereas others find no support for a direct link between the use of genetic engineering crops and yield increase.10 Even if these last conclusions were not accurate, the role of GM crops in combating famine remains questionable if not accompanied by adequate social choices.11 Indeed, the increase of food production does not guarantee access to foodstuff. This holds in the case of conventional breeding techniques as well.
For example, evidence shows that the number of hungry people during the ‘green revolution’ went from 536 million to 597.12 FAO studies give further proof of the increased poverty. In 2010, the number of hungry people was 925 million. This represents an increase of 75 million compared to the period 2006–2008. There is no question that poverty and distribution issues are at the heart of the problem of access to food.13 This, nevertheless, does not diminish the potential of plant breeding to fulfill the dietary needs of human beings. In this regard, the effects of GM crops on human health are surrounded by significant controversy. The most contended aspect is the risk of transferring allergens from one organism to another. This was the case of people allergic to nuts who reacted to GM soybeans into which a protein from a Brazil nut had been inserted.14 Health fears combined with several institutional and social factors have led to a strong movement of opposition to the agricultural applications of GM crops.15 This hostility is more perceivable in European countries where only a few GM organisms have been authorized for cultivation and use in aliments.16
3.1.2 Plant Breeding and Biodiversity
The Convention on Biological Diversity defines the term biodiversity as ‘the variability among living organisms from all sources including, inter alia, terrestrial, marine and other aquatic ecosystems and the ecological complexes of which they are part; this includes diversity within species, between species and of ecosystems.’17 This definition presents a unified view of the three levels in which biodiversity has been traditionally identified by scientists: genetic diversity, species diversity, and ecosystem diversity.18 Although a thorough description of these biodiversity levels and their relation with plant breeding would be interesting, it goes beyond the scope of this work. What matters most here, is to understand the importance of biodiversity as a whole, and if and how, plant breeding plays a role in biodiversity enrichment.
The value of biodiversity is strongly linked to the potential and the unknown importance of genetic variation. This uncertainty on the actual and future values of genetic diversity requires the maintenance of a broad base of biological variation. Two are the main reasons to be recalled: the possibility of dramatic events that could extinguish particular crops as a result of pest infestations or diseases, and the benefits associated with retaining a large number of genes. The former requires biodiversity because presence or absence of a single gene may be determinant to provide resistance to such threats. The latter, instead, relies on biodiversity to generate an incremental improvement or adaptation of different combination of genes, which is mainly dependent on the large numbers of genes.19
This understanding of biodiversity became a particular concern after the introduction of modern varieties, which present greater genetic uniformity. Two are the most worrying consequences of genetic uniformity: increase of vulnerability to pest and diseases, and the replacement of traditional landraces. The increased vulnerability to pest and diseases does not mean that modern crops are more vulnerable than traditional ones, but that harmful effects of a potential pest attack significantly increase in case of genetic uniformity. This is mainly because uniform genes offer a greater base which can be successfully attacked. The Southern Corn Leaf Blight—which reduced by 15 % US corn yields in 1970—is a significant example in this respect.20 Pest infections and diseases, however, have characterized agriculture production since its very beginnings. Creation and destruction of life forms are part of nature life cycles. In this regard, the merit of modern plant breeding has specifically been that of providing a stable release of varieties with greater resistance to pest and diseases.
Likewise, the gradual replacement of traditional landraces with new uniform varieties forms part of biodiversity concerns. FAO estimates that since the 1900s, some 75 % of plant genetic diversity has been lost as farmers worldwide have left their multiple local varieties and landraces for genetically uniform, high-yielding varieties. Today more than 90 % of crop varieties have disappeared from farmers’ fields. Moreover, of the 4 % of the 250,000–300,000 known edible plant species, only 150–200 are used by humans.21 There is no question that these figures offer proof of the disturbing effects of the genetic uniformity of modern varieties. What should be questioned in this respect are the methods used to measure biodiversity. Indeed, there are various measures for genetic diversity. According to the special reasons for studying biodiversity, biologists, ecologists, plant breeders, farmers, policymakers, and economists use different criteria to measure biodiversity. Obviously, this leads to different results. Even more significantly, it is not possible to identify a measure that fulfills all desired criteria.22
Thus, if we measure biodiversity by the number of landraces at country level, some studies show the decrease of genetic variation, while others give proof of diversity conservation. In 1935, for example, Germany witnessed a sharp decrease of the number of landraces of wheat varieties from 454 to 17 accepted cultivars and 54 accepted without reservation. On the contrary, a recent study showed an increase of diversity over time in wheat in Germany.23
Concerns on biodiversity conservation, however, have become less urgent after the introduction of in situ and ex situ preservation methods.24 The in situ approach maintains biodiversity in its original habitats, i.e. farm fields, whereas the ex situ approach conserves biodiversity in areas different from their original habitats, such as zoos, botanical gardens, and germplasm banks. The access to these germplasm collections facilitates the work of plant breeders to keep ahead of pest and diseases through a continuous flow of genetic diversity. Facilitated access to genetic resources for breeding purposes has further been promoted by the Multilateral System for access and benefit-sharing provided by the International Treaty on Plant Genetic Resources for Food and Agriculture (ITPGRFA).25 The Multilateral System involves all Contracting Parties, the international ex situ collections of the Consultative Group on International Agricultural Research, national agricultural research institutions26 and, additionally, encourages the participation of the seed industry.27 Given the role and the interest of the seed industry in a broad base of genetic resources, a meaningful and proactive participation of private firms shall be recommendable.28 This would create a balanced participation of all stakeholders as well as contribute to their common goal of expanding the base of available genetic resources in view of better yields. Finally, it is worth noting that balancing high and quality yields with biodiversity conservation techniques should be the main concern of plant breeding policies in order to benefit from science.29 The use of science, indeed, can be a double-edged sword when it is applied with no or little understanding of it. On the contrary, a well-informed comprehension of both negative and positive aspects of new technologies helps us shape future progress.
3.1.3 Plant Breeding and Sustainability
Since the Brundtland Commission of the United Nations introduced the concept of ‘sustainable development’, the term ‘sustainability’ has been used to indicate human activities on the environment.30 Thus, sustainable development indicates that type of ‘development that meets the needs of the present without compromising the ability of future generations to meet their own needs’. In the context of plant breeding, this means that the creation of new varieties should aim at the satisfaction of present needs while at the same time preserving genetic resources for future generations. This task is particularly challenging nowadays as climate changes are continuously depleting vital resources such as land and water.31 The exhaustion of natural resources in concert with population growth imposes the necessity to produce more food with fewer resources. The question is how and what can plant breeding contribute in this regard.
The approach taken by plant breeding to cope with these needs addresses in particular strategies of adaptation to climate changes.32 This is especially important in areas where climate change increases the frequency of abiotic stresses such as heat or draught, the probability of biotic stresses like pest and diseases, and causes the loss of biodiversity. Having widely discussed biodiversity issues in the previous paragraph, emphasize will be put here on the role of plant breeding in tackling climate change issues.
The role of plant breeding in climate change through the production of improved varieties has been astonishing. For instance, Bangladesh breeders have developed a new type of rice (Sub1) tolerant to flood that can survive total submersion for more than 2 weeks. Another example is that of beans varieties developed to tolerate the heat of the hot Durango region in Mexico and the cold high altitudes of Colombia and Peru.33 Recently, non-hybridized crops have given the same results. Scientists from the International Maize and Wheat Improvement Centre (CIMMYT) and the Kenya Agricultural Research Institute (KARI) developed maize seed varieties that yield well with minimum soil moisture. This type of maize is currently being adopted by smallholder farmers in Kenya’s Eastern Province.34
In addition, the modern techniques of breeding require less land for cultivation due to the increased productivity of new varieties. This was confirmed in a study on grains and oilseeds.35 Another stunning prospect is that of GM eucalyptus trees engineered to grow 20–30 % faster for use as paper, as pellets for power stations and as fuel for cars.36 These examples make apparent how important plant breeding is for meeting sustainability criteria. By producing high-yielding and high-quality crops, plant breeding guarantees a continuous supply of varieties in the present, and at the same time responds to the needs of future generations by paying special attention to environmental and climate change issues.
3.1.4 Plant Breeding and Economic Growth
Plant breeding does not only contribute to our human survival, but contributes to the wealth of countries as well. North America was one of the first countries to recognize the fundamental role of the import of plant varieties in trade. During the nineteenth century, the consular and naval personnel largely supported the import of plant germplasm from every part of the globe. For example, the Perry naval expedition of 1853 opened the harbors of Japan to American trade.37 The large base of germplasm acquired in foreign countries allowed American seed companies to create improved varieties, which were afterward exported in other countries. Extending trade with these other countries was particularly advantageous for the flourishing of the American seed industry.
The benefits of plant breeding for national economies, however, became better apparent after the Italian agronomist and plant breeder, Nazareno Strampelli, crossed different varieties of wheat to obtain new hybrids. This method proved to be more effective than the traditional selection of seeds within a single variety.38 The production of hybrid wheat, thus, allowed Italy to go from an average yield of 1.0 t/ha at the beginning of the twentieth century to about 1.5 t/ha in the 1930s.39 Thanks to Strampelli’s work, Italy did not only become self-sufficient in wheat production, but could also export wheat varieties in foreign countries. Indeed, the wheat varieties exported in Mexico put the basis for the ‘Green Revolution’ started by Norman Borlaug.
Since this period, international seed trade has constantly increased. In 2012, United States followed by China and France was the leading country in the global seed market.40 In 2010, seed imports and exports for flower seed, vegetable crop and field crop seed were dominated by countries such as US, the Netherlands, France and Germany.41 Figure 3.1 plainly illustrates the growth of the global seed market.
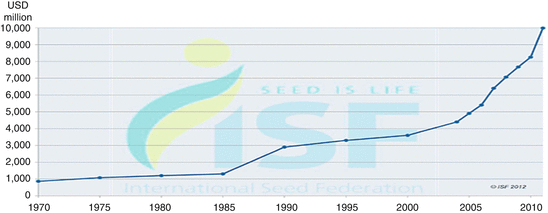
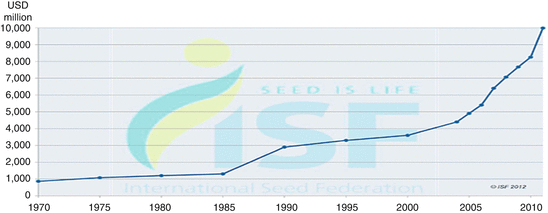
Fig. 3.1
The growth of the global seed market from 1970 to 2010
The role of the seed industry in trade becomes apparent in the figure. Since the beginning of the Green Revolution in 1970, the improvements in plant breeding techniques and production have been accompanied by a steadily increasing trade. Being trade a key factor in the development of countries, the benefits deriving from it are directly perceived by the society.42 For instance, trade might contribute to poverty alleviation.43 Vietnam is an example in this respect. After increasing production and exportation of coffee of 15 % in 1990, the agricultural GDP grew 4.6 % per year. This was accompanied by a reduction of rural poverty from 66 % in 1993 to 45 % in 1998.44
Plant breeding represents a vital industry for the economies of industrialized countries as well. In the Netherlands, for example, between 8,000 and 10,000 employees were estimated to work in the plant breeding and propagation industry for the year 2009.45 Moreover, increased yields enable countries to be self-sufficient in crop production. An illustrative case is the reversal of United Kingdom’s historical dependence on North American wheat imports.46 The benefits of plant breeding, therefore, are apparent in the economic development of countries besides food production and environmental sustainability.
3.2 The Evolution of Plant Breeding: From the Farmer to the Industry
Plant breeding started as a non-commercial activity carried out by farmers whose primary objective was to satisfy the needs of the family and eventually those of the village. At that time, the breeding of plants relied on farmers’ knowledge and on a few unrefined farming tools. Farmers selected and crossed existing plants in order to obtain new varieties with desired characteristics. This idyllic vision of a single farmer cultivating its piece of land has been gradually overwhelmed by the flourishing of the industry of plant breeding. The success of modern plant breeding is mainly due to biotechnological discoveries which enabled the creation of new breeding tools.47 These new techniques facilitated access to genetic resources of wild and unadapted varieties.
As a result of these discoveries, two main types of processes can be distinguished in plant breeding: conventional breeding and genetic engineering. Conventional breeding is performed by breeders who manipulate the sexual recombination process through selection and crossing of plants and their offspring in order to obtain a variety with desired characteristics. Whereas genetic engineering is carried out by biotechnologists in laboratories who modify plant genotype by inserting a genetic material from another organism (transgene). The ‘transgene’ may, for example, control for a specific trait and alter the original genotype of the plant and render it ‘transgenic’.48 Both conventional breeding techniques and new plant breeding techniques are necessary components of the so-called ‘plant breeders’ toolbox’.49 Instead of replacing traditional breeding methods, the new plant breeding techniques further enriched them. In specific, the development of herbicide tolerance and insect resistance traits is deemed to serve as proof of the introduction of the new methods of plant breeding. The most recent breeding techniques are able to modify the genome of plants by inducing mutations through epigenetics50 or inserting genes in a permanent or transient way.51
The first appearance of modern breeding techniques followed the discoveries of Darwin and Mendel in genetics. Mendel, in particular, provided a first explanation of genetic inheritance in the mid-nineteenth century. However, it was not until the early twentieth century that his work was recognized as the basis of modern plant breeding. Genetic discoveries allowed plant breeders to advance the understanding of plant genetics and enabled them to identify specific genes responsible for particular functions or attributes in a selected variety. This made possible a better adaption of plant varieties to human needs. The importance of this scientific evolution was first realized by public research institutions and universities which carried out basic research and delivered breeding products as public goods to farmers.52 This put the basis for the initiation of commercial plant breeding.
The potential profit deriving from the application of biotechnology in a business environment made crop research and breeding more appealing to the private sector. Additionally, the private sector had the knowledge and resources necessary for commercializing the products resulting from new technologies. However, business firms initially lacked scientific knowledge. It were highly motivated academic entrepreneurs that carried out the scientific knowledge into the commercial sector through the creation of new firms.53 Subsequently, breeding products acquired a greater economic value and gradually started to be treated as private goods and protected through various forms of intellectual property. Since then, R&D investments in agriculture have witnessed ‘an ebbing public sector and a rising private sector, aided and abetted by changes to IP’.54
3.2.1 Industrial Plant Variety Production
The breeding and commercialization of varieties goes through a long process that comprises four phases: formulation of breeding objectives, creation of variation, selection, and testing and finalizing varieties for the market.55 The formulation of breeding objectives is, therefore, the phase that precedes variety production by setting the type of research and breeding to be conducted for the final variety. For genetically modified (GM) varieties, an additional middle phase can be distinguished between the first and the second one. This phase consists in conducting research and tests finalized at demonstrating that the variety containing genetically modified organisms is safe for environmental, food, and feed use. Environmental, food, and feed safety—or the so-called—biosafety assessment, is to be demonstrated in compliance with national and international rules.56 These rules apply strict requirements which make this process very costly as well as lengthy. Producing a GM variety or applying a GM breeding technique is additionally expensive because the registration costs are particularly high for techniques classified as GMOs.57
Once a plant variety is finalized, it should comply with additional regulations in order to establish the value of the final variety. To this purpose, all crops, vegetables, and ornamentals are required to fulfill three requirements: distinctness, stability, and uniformity (DSU).58 Varieties that do not comply with the DSU requirements cannot be registered in national catalogues and therefore, cannot be commercialized.59 For field crops, there is an additional regulation: field trials have to be carried out in the whole country to ascertain the performance of the crop. These tests are deemed to be very useful in plant breeding since they stimulate the development and selection of the best varieties. The costs for this testing are particularly high for genetically modified seeds, which undergo a complex procedure of detection, identification, and quantification of specified traits.60
Breeding of new varieties is thus a complex, lengthy, and costly process. Although new and improved technological tools have recently enhanced the speed and accuracy of the breeding process,61 the costs of breeding and marketing plant varieties have not significantly changed. It has been estimated that seed companies invest approximately 12–15 % of their annual turnover on research and development,62 and breeding times for plant varieties may take from 7 to 15 years.63 Research expenditures, nevertheless, do not guarantee success in the market place. Only a favorable outcome of seed testing determines whether the plant variety would enter the market. Above all, the success of further commercialization depends on the ability to respond to the market demand. Considering the length of breeding processes, this means that firms should anticipate costumers’ preferences from 5 to 10 years in advance. Moreover, consumers’ preferences on GM or non GM varieties further constrain breeder’s choices. Depending on the structure and business model of seed companies, this type of decision may have an impact on its annual financial returns.