Representing Climate Change: Science, Social Science, Interdisciplinary Approaches and Lived Experience
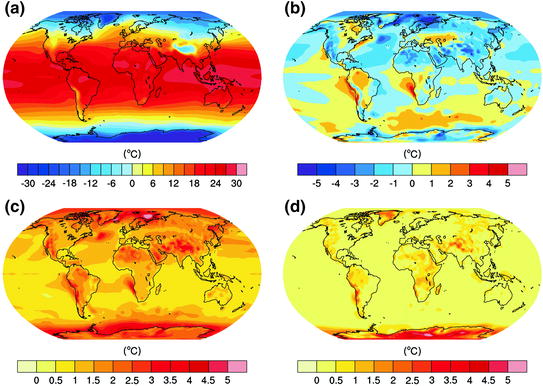
1.
Have a relatively well-defined and stable problem statement.
2.
Have a definite stopping point, i.e. we know when the solution is reached.
3.
Have a solution which can be objectively evaluated as being right or wrong.
4.
Belong to a class of similar problems which can be solved in a similar manner.
5.
Have solutions which can be tried and abandoned.
6.
Come with a limited set of alternative solutions.
Table 4.2
The attributes of ‘wicked problems’
1. | There is no definite formulation of the problem. The problem includes a permanent feedback with its environment. The process of formulating the problem is interconnected with the process of its solution. To ask all the relevant questions to gain information on the problem, you must know all the conceivable alternative solutions. |
2. | There are no stopping rules. The logic inherent in the problem does not tell you when to stop the inquiry. |
3. | There are no criteria for correctness. There is nothing in the problem to say how the solution should be judged. |
4. | There is no immediate test of the quality of the solution. |
5. | There is no ultimate test of a solution. |
6. | Once committed to a plan of action, change is consequential. You cannot make consequences not happen. |
7. | There is no list of permissible operations (If you have a habitual set of actions or prescriptions, try to break out.). |
8. | There are no well-defined solutions. You either have many solutions or none. The probability that a wicked problem has one solution is null. |
9. | Every wicked problem is unique. |
10. | The problem solver has no right to be wrong. Designers are responsible for their work. Unlike scientists, they do not put up work for rejection at a later time. |
In summary, a ‘tame problem’ may be technically complicated but have a relatively achievable and stable solution.
As Rittel and Webber (1973: 161) argue, ‘The classical systems approach … is based on the assumption that a planning project can be organised into distinct phases: ‘understand the problems’, ‘gather information’, ‘synthesise information and wait for the creative leap’, ‘work out solutions’ and the like. For ‘wicked problems’, however, this type of scheme does not work. One cannot understand the problem without knowing about its context; one cannot meaningfully search for information without the orientation of a solution concept; one cannot first understand then solve’.
‘Wicked problems’, on the other hand, are neither true nor false, they have no complete resolutions, they have no moral implications (as maybe the term suggests), but are as complex as the world we live in. There is often little or no consensus to either what the problem is or how to resolve it, and if anything, ‘wicked problems’ are forever dynamic and active in evolving even newer social dimensions and contexts, coming to life in our attempts to identify the problem itself, rather than solve it!
Within a world full of ‘wicked problems’, climate change is a ‘super wicked problem’ according to some (Lazarus 2009: 1153). Thus, in asking whether science can fix climate change, Hulme (2014: 119) argues that wicked problems of climate change defy ‘rational and optimal solutions…. And need to be approached differently, obliquely, if its dangers are to be defused’. But how do we do this? Climate change science is still dominated by natural, especially physical, scientists but it is true that there are now considerable inputs to knowledge from the ‘humanists’ or social scientists, for example in making us aware of the actual and potential human and societal impacts of climate change.
It is increasingly clear that the enormity and complexity of climate change cannot be addressed within singular disciplinary modes of inquiry, especially if we expect people to relate to and act in response of climate change knowledge. We need to take into account: (a) that there are several stakeholders from differing interest backgrounds, each with valid perspectives, (b) that the more the contribution from various disciplines across the natural and social science disciplines, the more the chance of constructing a wider knowledge base dealing with the layers of complexities embedded in ‘wicked problems’, and (c) lived experiential knowledge may contribute significantly to this wider knowledge base.
4.3 Fundamentals of Natural Science Inquiry and the ‘Scientific Method’
‘Science’ is a broad category which includes ‘natural’ as well as ‘social’ science. Natural science embodies those fields of knowledge that deal with objects and processes in the ‘biophysical world’—an enormous spectrum that includes, inter alia, the entire solar system within the Milky Way galaxy, the smaller and varied scale atmosphere that surrounds our planet, the geological formation of oil-bearing rocks beneath the sea bed, a colony of mountain gorillas, or even the inside of a wasps nest or termite mounds.
We have traced, in Chap. 3, the fundamentals of scientific inquiry back to the ancient Greek philosopher Aristotle. Here we delve more deeply into natural scientific inquiry. Underpinned by a ‘naturalistic approach’, such inquiry seeks reliable knowledge through measurement, testing and interpretation. It revolves around the five stages of a ‘scientific method’ as described by Russell’s 1931 (p. 57):
(i)
First is ‘observation of the significant facts’, leading to questions such as, Why did that do that? How does that work? What effect does that have? What are the processes that makes that happen? Or indeed, How can I solve that problem?
(ii)
Second involves ‘arriving at a hypothesis’ which if positive, will account for the natural phenomenon under observation;
(iii)
The third stage is to ‘deduce from this hypothesis consequences which can be tested by observation’ for prediction;
(iv)
The fourth stage includes designing and undertaking observations or experiments to prove or disprove the hypothesis; and
(v)
The final stage involves writing up and dissemination of results, publicly disclosed for other scientists to read and replicate.
‘Scientific method’ is thus characterised by empirical facts gauged through direct observation; systematic, careful and rigorous planning of test observations or experiments; control checks and replication to confirm the reliability of the result; and verification of the work to prove validity. Many scientific tools and techniques are used to support observation and experimentation with frequent use of those borrowed from complementary and overlapping ‘formal sciences’, for example mathematical and computer modelling, and moving towards proof of a hypothesis.
This quest, as embedded in the underlying philosophy of the ‘scientific method’ is defined in two key elements, (i) the critical role of empirical evidence, and (ii) the significance of ‘laws’ of physics, chemistry and other science subjects. An important requirement and a basic assumption of ‘scientific method’ is that all hypotheses and theories must be tested systematically against observations of the natural world and framed within the laws of pure sciences. Hence any claim to scientific knowledge has to be verified through observations, measurements, experiments, data collection. In fact, this is the only valid evidence, and anything that is intuitive or instinctive requires ‘objective’ verification through rigorous empirical testing—a notion that is increasingly challenged by social scientists as discussed later in this chapter.
The fundamental argument behind the scientific method is that science should only be concerned with the natural world and ‘facts’, and that all hypotheses and theories must be tested against observations of the natural world, measured or observed by an objective researcher in order to justify new ‘truths’. This fundamental argument is embedded in the principle of empiricism. It invokes that knowledge is obtained through experience that is acquired from evidence obtained especially through experimentation and which is underpinned by some manner of sensory awareness in its various forms, depending upon the disciplinary strand. For instance, experience and sensory awareness may mean different things for a psychologist compared to a physicist. However, whatever the difference, empiricism considers observation and evidence as fundamental to the establishment of ‘truths’. Thus for instance, we moved several centuries ago from the historical ‘truth’ that the Earth is flat to one that the earth is close to a sphere, a truth that was originally supported by natural ‘facts’ such as the movement, or otherwise, of the sun/moon, and today is supported by observations from satellite images.
Natural scientists also endeavour to create theoretical explanations based on causal relationships that are determined by universal laws, meaning that, wherever tried and tested, the result is the same. Examples include the theory of gravity, the behaviour of hot and cold air and so on. To defend laws and theories as ‘knowledge’, scientific method turns to positivism. A positivist evolution is associated with the early 1830s French philosopher Auguste Comte who challenged notions of speculation and utopian ideas of pre-revolutionary France in what he termed a ‘negative philosophy’. Instead he argued for a positivist view based on the real world of given circumstances and material objects. Thus positivism focuses on ‘positive’ facts and actual, rather than imaginary, observable phenomena. In doing so, the approach avoids metaphysical questions (such as those related to being and self) and theological questions (about God) that dominated the era, and which could not be measured scientifically.
A positivist rationale therefore embraces careful measurement and objective data collection to create general laws to predict and explain nature. To obtain objective data, interference from the researcher’s personal or political values, which may mar ‘truths’ and knowledge, needs to be kept in check. Positivist knowledge thus requires propositions or hypotheses and laws and theories to be tested and verified, hence it provides the foundation of ‘scientific method’. In fact, pure positivists are so sure about the ‘scientific method’, they argue that this is the only methodology that can capture the true essence of natural inquiry which may be applied universally. Since Comte’s original formulation, positivism has evolved and divided into several strands, two of which are summarised in Box 4.1.
Box 4.1 Logical positivism and critical rationalism
There are several contemporary versions of positivism, with two historically influential ones being (i) logical positivism and (ii) critical rationalism. The roots of logical positivism are in the 1920s Vienna positivists who argued that theories are only meaningful if they could be tested and verified—and this included theories beyond the realm of natural science, for instance, social science theories. Thus they turned to logic to make ‘scientific method’ more rigorous for verifying theoretical ‘truth’ or falsity of empirical statements. For them, verifying or falsifying requires propositions to be justified against reality, without resorting to experiential analysis or synthetic statements whose ‘truth’ needs to be established by empirically tested hypotheses. Logical positivism attracted some important names amongst whom are early logicians such as Ernst Mach and Ludwig Wiggenstein and later Bertrand Russell. Whilst logical positivism contributed significantly to the philosophical debates of logic, language and mathematics in their day, this ‘totalising’ approach has since been discredited as over-simplistic. We may, however, perceive overtones of logical positivism persisting among many climate scientists, where the validity of models that predict the future under global warming scenarios is established through how well they also describe contemporary and historical climates (see also Chap. 2). As the IPCC 5th Assessment Report for Working Group 1 states: ‘The direct approach to model evaluation is to compare model output with observations and analyse the resulting difference’ (IPCC 2013: 746).
A challenge to logical positivism came from the critical rationalism of Karl Popper, also a philosopher, in the 1930s. Critical rationalism, as the name implies, is about reflecting on claims to knowledge in a critical and rational manner. To do this, we can only accept a claim that can be proved and/or through reason and experience. Popper argued that empirical evidence cannot be confirmed in advance of the event and thus it is possible that we may come across inconsistencies. Therefore, if the claim is based on empirical findings, it can be tested in order to falsify it. He argued that in testing a theory or other claims, scientists are in fact not looking for evidence, but counter-evidence to see whether or not the empirical evidence is refutable or false. Contemporary critical rationalism elaborates Popper’s arguments to several areas of thinking and action around particular problems, fundamentally to replace justification methods with critical rationalism. The United Nations Intergovernmental Panel on Climate Change (IPCC) process, that reviews and assesses the most recent scientific, technical and socio-economic information produced worldwide relevant to the understanding of climate change, may be seen as an exercise in critical rationalism (see Chap. 3, Box 3.4).
Critical underlying assumptions of natural science are thus partially built into the fundamentals of a ‘scientific’ methodology and partially on a positivist philosophical rationale. The goal of inquiry is to explain and predict. Most positivists would also say that the ultimate goal is to develop the law of general understanding, by discovering necessary and sufficient conditions for any phenomenon (creating a perfect model of it). If the law is known, we can manipulate the conditions to produce the predicted result. In this, the essential premises of scientific inquiry comprise (i) both inductive (where research comes before theory) and deductive reasoning (the theory comes before the research); (ii) value free and objective research; and (iii) validity and reliability.
4.4 Underlying Assumptions of the ‘Scientific Method’ and Positivist Objectivity
A broad acceptance of the critical underlying assumption that ‘scientific method’ leads to objective, value free, neutral ‘truth’ untarnished by personal beliefs, bias or preferences has given natural science an extremely dominant position in society. Powerful institutions within which science has historically resided (such as the Royal Society in the United Kingdom and its equivalents in other affluent countries, and the Christian Church in Europe) have meant that throughout history human populations have been willingly or otherwise obliged to live by its truisms. This has especially been the case where dominant institutions have understood science to be in their service (even if this does mean that claims to be ‘objective, value free and neutral’ ring hollow). The alignment of science and the Christian Church, for example, ruled lives in the Europe of the Middle Ages (475–1550 AD) when the Church controlled all scientific and philosophical ideas about the natural world. Through this control, it established ‘undeniable truths’ about biology, physics and astronomy based on the Biblical interpretations and the teleological reasoning—meaning that everything is to be explained by the ultimate purpose it serves (Chap. 3, Box 3.2)—which was so distinctive of the philosophical system of the ancient Greek philosopher, Aristotle. This was a powerful doctrine. It was not until the ‘scientific revolution’ (circa 1550–1700) that new ideas in physics, astronomy and human anatomy began to challenge and undermine the laid-down laws and views of the church and the Aristotelian system of teleological reasoning. Two publications in particular were seen as the tipping point for the start of the revolution: Nicolaus Copernicus’s De revolutionibus orbium coelestium (On the Revolutions of the Heavenly Spheres) which shifted from the geocentric model and placed the sun at the centre of the planetary system and Andreas Vesalius’s De humani corporis fabrica (On the Fabric of the Human body) which challenged existing Galen’s theory of humours through observation and meticulous drawing of the human body, its skeleton, muscles and bones. Science carries this legacy of establishing ‘truth’ based on an ‘objective’ method of inquiry even today.
Notwithstanding recently documented evidence of falling public confidence in science (see Sect. 4.6 below and Chap. 7), it has also historically been, and largely continues to be, dominant in that it is perceived to work, that is, it provides solutions to problems—it delivers the goods. This might be through direct application, such as in engineering, but also through providing evidence for policy, as does climate science. The issue here then does not ultimately concern science, but the definition and representation of the societal problems that it is marshalled to solve?
To give one pertinent example, associated with the continuing growth of a capitalist, industrial society mainly through Europe and the United States in the late nineteenth and early twentieth century was a continuing problem of finding a more effective mode of assisted transport than a horse and cart, and which was more flexible than the steam railways. The internal combustion engine, based on the scientific laws of thermodynamics, was invented in 1868 by the German, Karl Benz and led to the automobiles that we know today. Secondary impacts were conceived only through comparison of the automobile with the horse which it sought to replace, the former appearing much cleaner and easier to maintain. With the advent of the automobile, the smell and general health hazard of horse dung throughout the streets of London and other big cities would become history. Nobody at the time asked about the possible health hazards arising from pollution of the atmosphere through automobile emissions of lead, noxious gases and particulate matter, or if they did, which might have been the case in sci-fi novels, nobody took them seriously. Moreover, nobody wanted to take them seriously as, with increasing scientific knowledge, the future could look after itself. Certainly nobody asked whether increasing carbon dioxide in the atmosphere from internal combustion engine emissions might contribute to potentially harmful global warming.
The issue, therefore, does not always concern the power of science in public institutions per se, but the problems it is asked to solve. These are not necessarily completely wrong problems, but incomplete problems in that they have been defined in function of the imperatives of dominant societal concerns of their time. The ultimate power lies with those who are able to represent those dominant societal concerns—at the turn of the nineteenth/twentieth century the capitalist leaders of industry supported by the political class.
Whether science is seen to work or not, there are, however, several ways in which both the ‘scientific method’ and the ‘truths’ thus established may be challenged. To begin with, take the notion of objectivity—ultimately devoid of moral and political values—as central to empiricist and positivist approaches in natural science. To generate a research process that is rigorously designed and controlled, the researcher is expected to observe, record and analyse empirical findings neutrally at every stage in order to discover universal laws and ‘truths’. This does, however, beg the question of whether knowledge can ever be obtained in such a detached, objective and neutral manner. Every scientist is human, and no doubt has personal opinions, things in which they believe or disbelieve as individuals, their own favourite topics and interests which influence how they approach and see the world, all of which influence the questions they ask. This is clear, for instance with ethical dilemmas when doctors with strong personal moral beliefs will not research on human embryos. So, whatever the underlying philosophy and a legacy of how they or their mentors should carry out ‘objective’ research based on ‘objective’ criteria, it seems likely that internalised beliefs and a ‘human factor’ will still have a leading influence.
As societal creatures, we inevitably operate in a cultural environment that holds certain beliefs and doctrines, and researchers are not exempted from that! For instance as mentioned above, historically religious, absolute ‘truths’ dominated scientific thinking and processes in the Middle Ages. More recently, biologists have measured intelligence and head sizes in establishing ‘truth’ and evidence of racial inferiority and supremacy within their own cultural environment. Fortunately this theme has now faded (although not completely disappeared) from popularity. Further examples include the work undertaken by scientists working for the tobacco companies in the United States who have sought to discredit the scientific link between smoking and cancer, and those scientists who have questioned the link between anthropogenic carbon dioxide emissions and climate change. Arguably, because large multinational corporations have often funded much of this research, it has focused on finding a negative link rather than a positive one. Therefore, however hard scientists seek objectivity, many factors including who is paying the piper and the cultural context of the time within which human values are defined, impact on how hypotheses, research design and questions are represented and analysed.
A second but equally important assumption is based on the issue of validity and reliability. All good science aims to be valid and reliable. In natural science, valid research is that which accepts an empirical or positivist approach as discussed above, accepts the entire experimental concept and establishes whether the results meet all of the requirements of the ‘scientific method’. We should note that there are two aspects of validity to consider, internal and external validity.
Internal validity dictates how an experiment, observation or survey is designed. Internal rigour is an absolute necessity if valid results are to be produced. This requires an ordered and careful approach to data collection. External validity is further down the line and is concerned about how one processes the results and asks questions of them to discover causal relationships. These relationships are easier to validate if there is some form of control built into the experiment, for example the use of statistics including randomised samples or locations to determine significance and difference. Note the term ‘significant’ is not necessarily evidence of an ‘absolute truth’. Also correlation does not prove a relationship because there may always be some other reason, not yet measured or observed, which has contributed to the results.
Reliability refers to repeatability, comparison and consistency of a result. It is reinforced when other researchers are able to conduct exactly the same experiment, observations or surveys, under the same controls and obtain the same results. The use of instruments for measuring, for example, a stop watch, is also seen as a robust way to collect accurate and reliable data. In natural science, following a deductive approach, we can say that the more repeatable and consistent the finding, the more weight it adds to a hypothesis which eventually perhaps becomes recognised as a scientific law or ‘truth’. As noted in Box 4.1 above, climate change models, for example, are tested against past climate data to check for validity and reliability and many modifications are on offer on how to improve them. See Fig. 4.1 where the IPCC (2013: Chap. 9, Fig. 9.2) checks the accuracy of an ensemble of climate models against historical observed temperatures. Note for our purposes here that the relevant Figure is (b) where the shading represents the difference between temperatures derived from the ensemble of models and those observed. Mostly this is 1 or 2°, with exceptions especially around the polar regions where good data are less readily available. Yet, of course, none of this accounts for social and cultural contexts where reliability is gauged in other ways—through, for example, our lived experiences.
< div class='tao-gold-member'>
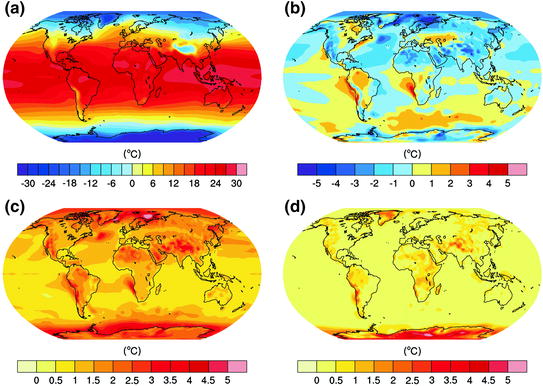
Only gold members can continue reading. Log In or Register a > to continue