Adaptive Management for Novel Ecosystems
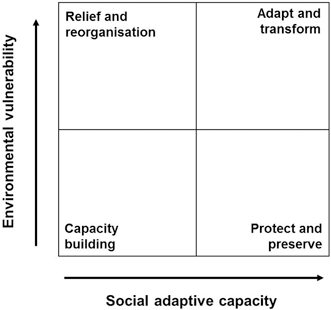
Fig. 8.1
Species of corals and fishes display differential susceptibility to a range of disturbances. A Acropora corals are highly susceptible to the effects of ocean warming, and some other threats, such as crown-of-thorns starfish outbreaks and storm damage. B Porites corals are much more tolerant of warm water and can often persist on reefs when many other species are lost. C Many species of butterflyfish are vulnerable to coral loss or a change in the composition of coral species due to their reliance on certain species of corals for food and/or shelter. D Species of grouper are vulnerable to population depletions through fishing because they are targeted for their size, and have life histories that make population recovery difficult. Photos A and B taken by N. Graham. Photo C taken by M Pratchett. Photo D taken by F. Januchowski-Hartley
The influence of these benthic changes on associated reef fish assemblage structure is also predictable, with susceptibility dictated by specialization and body size (Wilson et al. 2006, Graham et al. 2011). Species with smaller body size or high levels of specialization (diet, habitat use or settlement cues) are most vulnerable to reductions in live coral cover, or shuffling of the coral species present (Pratchett et al. 2008) (Fig. 8.1). Conversely, larger bodied species and those with slower life-history traits (such as slow growth rates and late maturity) are most vulnerable to fishing impacts (Fig. 8.1). Taken together, these non-random disturbance responses and recovery patterns of corals and fishes are likely to lead to predictable ecosystem configurations composed of different species abundances, compositions, structures and functions. Evidence supporting this notion is mounting, with large scale alterations to coral reef species composition persisting over extended temporal scales (McClanahan et al. 2007, Pratchett et al. 2011, van Woesik et al. 2011). Indeed, recognizing that ocean warming is leading to asymmetrical losses of species of corals, the novel ecosystem concept has been used to frame coral reefs of the Caribbean (Yakob and Mumby 2011), and more recently, the future of coral reefs globally (Graham et al. 2014).
These changing dynamics and compositions on coral reefs highlight that the pristine reef conditions of the past have become increasingly rare through time (Fig. 8.2). Indeed, it is highly unlikely that any reefs will exist in a completely pristine state in the future. The proportion of reefs that have undergone a shift to an alternative non-coral system state has increased through time, and is likely to increase more into the future (Fig. 8.2). However, the emergence of novel coral reefs gives hope for a future with some coral-dominated ecosystems, albeit with novel compositions. Indeed, the concept provides a much more pragmatic and attainable outlook on a future with coral-dominated reefs. Furthermore, local management actions may ensure a greater number of reefs are maintained in a coral-dominated novel condition, rather than in a degraded non-coral state (Fig. 8.2).
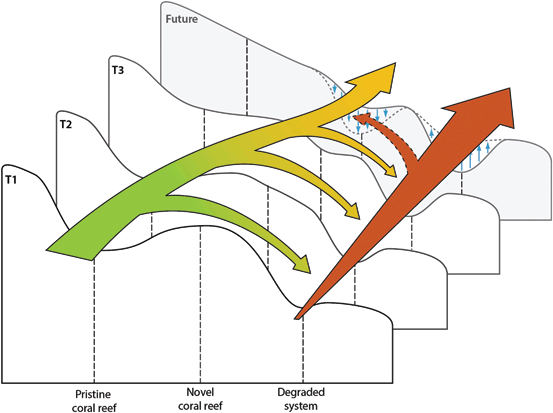
Fig. 8.2
Conceptualizing changing scenarios for coral reef ecosystems. Pristine coral reefs have become less common through time, and are unlikely to be a realistic goal for the future of coral reefs under continued human pressure and anthropogenic climate change . Degraded non-coral systems have become more common through time, and many argue will become the dominant reef condition in the future. However, a middle ground, where altered ‘novel’ coral reef community compositions persist may be a realistic goal for many of the world’s coral reefs. This will be possible due to the differential susceptibility of many reef organisms to a range of pressures. It may also be more likely with appropriate adaptive management actions, which reduce the number of reefs in degraded conditions and increase the number of reefs persisting in a novel condition (depicted by dashed lines and blue arrows in figure)
Will Current Management Paradigms Be Appropriate?
There is mounting evidence that local management can be important for coral reef ecosystem condition in the face of the growing global threats to reefs and may therefore help ensure more reefs are maintained in novel coral-dominated conditions in the future (Fig. 8.2). Although many of the major threats to coral reefs , such as temperature induced coral bleaching and tropical storms, are difficult to manage locally, two of the key threats (fishing and water quality) can be managed locally and doing so should provide substantial benefits to the ecosystem (Graham et al. 2013). Importantly, the functional groups of fish thought to be most important for reef recovery processes are responsive to local fisheries management policies (Graham et al. 2011). Well enforced marine reserves can lead to changes in ecosystem dynamics with increased fish herbivory, reduced fleshy algal cover and enhanced rates of coral recovery (Mumby et al. 2006, Stockwell et al. 2009, Mumby and Harborne 2010, Wilson et al. 2012). Furthermore, gradients of fish biomass highlight the importance of maintaining fish biomass above thresholds where key reef processes are reduced and the reef condition changes (McClanahan et al. 2011). Similarly, gradients or changes in water quality (sediments, pollutants and nutrients) highlight the potential for local reductions of negative terrestrial (catchment) input to enhance coral recovery and diversity (Smith et al. 1981, De’ath and Fabricius 2010).
Although compositions will be different due to the non-random reduction in occupancy, abundance, and recovery potential of species, local management should have the potential to stimulate continued recovery of hard coral communities into the future. If stewardship of reefs is improved and becomes adaptive to changing threats and social contexts, it should be possible to ensure a greater number of coral reefs are maintained in a novel ecosystem composition (Fig. 8.2). Critically, however, the unfolding novel composition of reefs highlights, more than ever, that we should not be complacent with our current management approaches. Instead, we need to re-evaluate how effective they are in novel coral reef contexts, and become more innovative in developing new adaptive management approaches that may yield new solutions, or alternative pathways to beneficial ecosystem configurations.
Marine protected areas have largely dominated the space for coral reef management. They are seen to be useful, partly because they represent measurable management outcomes based on the amount of area protected. Furthermore, implementing and managing marine protected areas can appear straightforward because they can be sited close to shore making monitoring feasible and are also amenable to top down enforcement and control. However, there is considerable debate as to the extent to which marine protected areas deliver conservation and fishery benefits (Sale et al 2005, Agardy et al. 2011, Mora and Sale 2011). In most countries no-take marine protected areas typically only represent a small proportion of the total coral reef area (McClanahan et al. 2008a), and compliance with the rules is often weak (Mora et al. 2006, Pollnac et al. 2010, Daw et al. 2011, Campbell et al. 2012). If compliance is not a large issue, marine protected areas can serve a vital role in the broader management of coral reef systems. However, less clear, and seldom articulated, is what that role is; and how, when, and where marine protected areas should be implemented. Marine protected area implementation would benefit from progressing beyond the precautionary principal approach that they currently fill (i.e., setting areas aside as an insurance) (Lauck 1998), to hypothesis driven implementation that is monitored and enables managers to learn what works and better anticipate realistic coral reef futures (McCook et al. 2010). Critically, although frameworks have been suggested (Grafton and Kompas 2005), marine protected area management on coral reefs is rarely adaptive, with rigid legislation locking an area of the ocean aside for goals that may end up changing though time, and against threats that may not be mitigated by closures. This highlights the need to manage coral reefs across wider reefscapes, reducing the negative effects of overfishing and other disturbances at larger scales (Steneck et al. 2009). The solution space needs to be more innovative, adaptive, and act at larger scales.
The Need to Manage for Uncertainty: Adaptive Management
The emergence of novel coral reef ecosystems and the recognition that species will have differential responses to a changing climate brings with it a growing urgency to manage effectively, for and with, uncertainty. However, the desire for management to be based on the best available science paradoxically hinders the very process it tries to advance. This is because science tends to be produced with laboratory style precision, leaving scientists reluctant to make recommendations based on partial information, and chastised when they do so (Willis et al. 2003). Management actions are therefore often delayed or developed in an ad hoc manner. This ad hoc management tends to be based on anecdotal evidence or advocacy and developed in response to emerging challenges and opportunities (Lee 1999, Willis et al 2003). Adaptive management offers a middle ground between laboratory style precision that tends not to be feasible and ad hoc trial-and-error that seems risky (Lee 1999).
Adaptive management is grounded in the admission that we do not know enough about managing ecosystems while simultaneously recognizing the need for action. Adaptive management further recognizes that ecosystems are naturally dynamic; and because they are inextricably linked to the human communities that interact and depend on them, they are also complex. Uncertainty is therefore inherent in any management decisions that are made regarding these dynamic and complex systems . Because a lack of information should not delay action, adaptive management explicitly addresses the uncertainties to present a structured process of learning by doing , and adapting based on what’s learned (Walters and Holling 1990). Implicit is an acknowledgement that the most important uncertainties should be tested early. By recognizing the value in tracking ecosystem responses to management, this approach enables management to proceed with impartial knowledge (Lee 1999). Strategies are developed as experiments to provide information about the system that is being managed, and this information is then used to refine, or develop, future strategies (Holling 1978, Walters 1986). Because adaptive management is designed to employ a diversity of testable actions, it affords a system a greater capacity to manage for resilience than the current tendency to employ single policies across ecological space or time that could potentially lead to disaster (Tompkins and Adger 2004, Bodin and Norberg 2005).
Adaptive Management and Coral Reefs
Effectively managing single marine fish stocks for economic return has proved challenging (Roughgarden and Smith 1996). Managing complex systems with multiple benefits, as is the case with most coral reef settings, is recognized as far more challenging. Although adaptive management has been suggested as an appropriate way to address these challenges, there are relatively few examples of adaptive coral reef management. The re-zoning of the Great Barrier Reef Marine Park in 2004 to incorporate a 33 % no-take area, rather than the previous 5 %, and protect a wider range of habitat types, is one of the few examples of an adaptive process (Hughes et al. 2007). This re-zoning was based on a huge amount of learning, including experimental fishing experiments in existing no-take zones (Mapstone et al. 2004, Sale et al. 2005), and a greater understanding of the functioning of the system. Ongoing understanding of the drivers of ecosystem change in the Great Barrier Reef is leading to renewed attempts to control water quality problems from adjacent catchments (Queensland Government 2009), and a great deal of science and management attention is currently trying to understand how to control outbreaks of predatory crown-of-thorns starfish (Rivera-Posada et al. 2013). Although this learning and management is clearly dynamic, whether changes to the zoning plan or water quality objectives will be possible at appropriate time scales is yet to be seen.
Customary coral reef management in parts of the Pacific has developed through processes of learning, experimentation and adaptation (Johannes 1982). For example, the use of periodic closures, where a section of the reef is closed to fishing to build up stocks and influence fish behavior (Feary et al. 2011) to ultimately enhance catch during important ceremonies or events, has been studied as an adaptive management cycle (Cinner et al. 2006). Indeed, the strong traditional ecological knowledge in parts of Melanesia has been harnessed to develop effective adaptive co-management arrangements between science NGOs and local communities (Weeks and Jupiter 2013). However, the huge changes occurring on coral reefs in response to climate change and other escalating drivers requires the adoption of adaptive management more widely and in a much more experimental way.
Experimentation, Monitoring and Learning
The core principles of adaptive management are that it is experimental, multi-scalar, and place-based (Norton 2005). Experimental recognizes the ongoing search for knowledge that is necessary to set and achieve goals. Multi-scalar stresses the need for managers to model and monitor management on multiple scales of space and time. Place-based accepts that ecosystems are occupied by people, therefore, the way people are likely to perceive and respond to management will reflect their social and geographic orientations (Norton 2005). The experimental component of adaptive management stresses the importance of not delaying action for a lack of knowledge and the opportunity to take advantage of the situation and learn from it. Management actions are therefore designed in a structured way such that lessons can be rigorously inferred, similar to the way in which experiments are designed. Management actions should therefore be designed so that they have clear hypotheses, a way of controlling factors that are (thought to be) extraneous to the hypotheses, and with opportunities to replicate the management “experiment” to check its reliability (Lee 1999). These management actions should be monitored so that the data provides a way of appraising the hypotheses, and can be continuously fed back into decision-making in a forward thinking way, rather than simply tracking toward some hypothesized ecological baseline. The emergence of novel coral reef ecosystems requires a great deal of experimentation and learning to elucidate appropriate management strategies to deal with changing ecosystem compositions and processes.
A great deal of scientific work is needed to fully understand the range of important ecological processes on coral reefs, how the relative importance of these processes may change in different contexts and with changes in reef compositions, and how we can effectively influence ecosystem processes in management. Most current attention is given to the process of herbivory, because herbivorous fish and urchins can maintain cropped algae that encourages successful coral recruitment and recovery processes (Bellwood et al. 2004, Mumby et al. 2007). Knowledge of herbivory on coral reefs is rapidly improving, with an appreciation of the non-linear relationships between body size and bite volume (Lokrantz et al. 2008) and foraging range (Nash et al. 2013). Furthermore, assessments of the feeding preferences of herbivores are identifying groups of herbivores that target turf algae to feed upon, and others that eat mature fleshy macroalgae (Bellwood et al. 2006, Hoey and Bellwood 2011). These different types of herbivore functions are key to influencing different reef trajectories, will vary according to reef condition (Graham et al. 2013), and may require different forms of management experimentation .
Although outright bans on catching herbivores have been enacted in some countries (Steneck et al. 2009), in other places innovative management experiments are being attempted such as escape gaps that allow key species of herbivorous fish to exit fishing traps (Johnson 2010, Mbaru and McClanahan 2013). Importantly, modifying fishing technologies in these ways can promote important ecosystem processes on coral reefs while maintaining the income of fishers (Johnson 2010). In other locations, phasing out certain types of fishing gear may reduce impacts on key groups of fish, as each gear is typically selective toward a certain portion of the fish community (Cinner et al. 2009a). Such restrictions on the types of gears in use may be used in experimental ways, or in response to specific impacts to reefs, in an adaptive management framework (McClanahan and Cinner 2008). For example, phasing out certain gears that target species of fish known to promote coral recovery post disturbance, may be an appropriate way to prevent reefs transitioning to potentially undesirable states (Cinner et al. 2009a, Graham et al. 2013). However, reducing catch of herbivores may not always be appropriate or ethical; in some heavily fished locations the dominance of herbivores is sustaining the catch of the fishery (McClanahan et al. 2008b, Hicks and McClanahan 2012).
Although the importance of the process of herbivory is well established in the ecology of coral reefs, other processes that have been largely overlooked may become increasingly important in understanding and managing reefs in the future. One example is the realization that excess sediments in algal turfs can exclude many species of herbivore (Bellwood and Fulton 2008). Moreover, these longer turf algal states with sediment loads can be a barrier to successful coral settlement and establishment (Arnold et al. 2010). As such, the process of sediment removal from reefs is likely to be important in many locations, but poorly understood. There are likely to be a range of other processes, such as excavation of dead unstable carbonate structures, which are poorly understood, and thus not incorporated into management actions. Improving this understanding will allow management experimentation to establish options for enhancing certain reef processes on reefs dependent on the condition and dominant processes required to improve reef trajectories.
Changes in reef community composition are likely to cause shifts in species dominance patterns, which resource users and managers should carefully evaluate. For example, lightly fished coral reefs where coral communities are not too heavily impacted may be able to sustain a fishery on reef predatory species, such as the coral trout fishery in Australia (Russ and Williams 1994). However, in locations where the coral reef community has shifted substantially, and the fishing is heavier, the dominant fish species on the reef and those that sustain the catch of local fisheries can be quite different. In these contexts, slow growing species of predators have been over-exploited long ago, and the catch composition represents a suite of species with faster life history traits (McClanahan and Hicks 2011). This may also interact with the condition of the reef. For example, in Kenya fishing pressure is high and corals in the lagoon area have become scarce, leading to a more seagrass and macroalgae dominated system. Over 60 % of the biomass of the catch along this stretch of coastline is made up by two species of fish: the rabbitfish Siganus sutor, and the parrotfish Leptoscarus vaigiensis (Hicks and McClanahan 2012). Both of these species feed on seagrass and mature macroalgae (Chong-Seng et al. 2014). Indeed, before-after studies in the region assessing changes in reef condition and fish assemblages in response to the 1998 coral bleaching event, showed that macroalgal feeding species of rabbitfishes and parrotfishes like Siganus argenteus, Leptoscarus vaigiensis and Calotomus carolinus were among the few species that responded positively to the disturbance (Graham et al. 2007). Importantly, some of these species have very fast life history traits, with the ability to grow to plate size and reproduce within 12 months (Hicks and McClanahan 2012). The opportunities for emerging, potentially sustainable, fisheries dominated by “new” species needs to be carefully monitored. Importantly, rather than management attempting to maintain fisheries of species that are being depleted due to local fisheries and slow life history strategies, or factors external to the fishery (e.g., loss of recruitment habitat), management that is adaptive can take advantage of more sustainable fishing opportunities when different species become abundant. Clearly, such decision making will need to navigate trade-offs between biodiversity conservation and food security/livelihood needs, and may require mixed management approaches across the seascape (McClanahan et al. 2011, White et al. 2012).
Although managing reef processes and adapting to changing dominance patterns in reef resources may be preferred and in some way optimal, in many situations it will be difficult to achieve. Setting more achievable management targets, based on scientific underpinnings with the flexibility to use a range of adaptive management approaches to achieve those targets may be one solution. Although fisheries have a history of single species targeting and associated population assessments for management, recent studies have suggested that a more balanced approach, where the whole fish community is exploited in a non-selective way, may be more appropriate (Zhou et al. 2010). Indeed, this premise has recently been applied to coral reefs , with the idea of biomass based fisheries management, which provides simple guidelines for how much fish biomass should be maintained on a reef to try to maximize both fisheries sustainability and ecosystem condition. Using a large ecological data set across the Indian Ocean, McClanahan et al. (2011) showed that as biomass is reduced a series of clear and abrupt changes occur in various reef processes and state variables, such as predation, switches in dominant herbivores and increases in fleshy algae. Using these ecological switchpoints, and principals of multispecies maximum sustainable yield estimates, they proposed a biomass window that resource users and managers in the region should strive for in order to promote functioning reefs and sustain fisheries. Importantly, a wide range of approaches may be employed to meet these targets, such as community closed areas, restrictions on the types of fishing gears that can be used, or enhancing the capacity to access pelagic resources, rather than reef-based resources. Management experimentation may be important in many settings to explore ways to achieve these biomass targets.
Moving toward a Social-Ecological Systems Understanding
Key to managing adaptively is having an explicit vision or model of the ecosystem one is trying to guide (Walters 1986). That explicit vision provides a baseline for defining surprise and thus expanding the boundaries of what is known (Lee 1999). A clear articulation of what novel ecosystems are likely to emerge is therefore critical to this process. Indeed, this has been put forward as a key research frontier for novel coral reef futures, with a range of methods available to achieve the goal (Graham et al. 2014). Understanding how to guide specific novel ecosystem emergence and enable desirable novel ecosystems to persist will be key to the adaptive learning process. Integral to the persistence of certain ecosystem configurations is the perceived desirability of the ecosystem goods and services that are delivered to the stakeholders that interact and ultimately benefit from the ecosystem. Understanding who benefits, how they benefit, and how they perceive those benefits is therefore also critical to the success of any management system (Lebel et al. 2006, Hicks 2011).
Changes in coral reef compositions are likely to affect the generation of ecosystem services, however few studies have explicitly investigated these changes (Graham et al. 2013). Dive tourists often desire high coral cover and lots of big fish (Williams and Polunin 2000), but less dramatic reef conditions, and even reefs dominated by soft corals, can still attract tourists. Reefs in different conditions will also likely offer very different fishing opportunities (Mumby et al. 2008). Although lightly exploited reefs, such as those in Australia, can support fisheries on high level predators such as coral trout (Russ and Williams 1994), in more heavily exploited and altered reef locations, lower trophic level species, such as herbivorous rabbitfishes and parrotfishes, can largely sustain the fishery (McClanahan et al. 2008b, Hicks and McClanahan 2012). Importantly, different stakeholders tend to value and prioritize ecosystem services in different ways (Hicks et al. 2013